The Impact of Pressure and Wind on the Landscape
The Nature of Atmospheric Pressure
Pressure—the force a gas exerts on some specified area of a container’s walls.
Atmospheric pressure—the force exerted by gas molecules in the atmosphere.
Affects Earth’s surface as well as any body on Earth.
Omnidirectional force—exerted equally in all directions.
Force decreases with increasing altitude because the actual number of gas molecules is also reduced.
Factors Influencing Atmospheric Pressure
The cause-and-effect relationship between these three variables is explained by the ideal gas law.
The Ideal Gas Law—the relationship between pressure, temperature, and density can be summarized by the ideal gas law equation: P = ρRT
P = pressure
R = constant of proportionality
ρ = density
T = temperature
The pressure of a gas is proportional to its density and temperature.
Pressure increases if the density remains constant but temperature increases.
Pressure increases if the temperature remains constant but density increases.
So, variations in any one—pressure, density, or temperature of atmosphere—affect the other two.
In a sealed container, changes are easy to determine; however, in the atmosphere, the relationship is very complex, so it is difficult to make exact predictions of how a change in one affects the others.
Density and Pressure Relationships
Density is the mass of matter in a unit of volume. The density of gas changes easily because gas expands as far as the environmental pressure allows.
The denser the gas, the greater the pressure it exerts.
Temperature and Pressure Relationships
If air is heated, the molecules become more agitated and they exert greater pressure.
Therefore, an increase in temperature equals an increase in pressure, and a decrease in temperature equals a decrease in pressure.
If a mass of air is not confined, it will expand when heated and may actually lead to a decrease in pressure as temperature increases.
Dynamic Influences on Air Pressure
Surface air pressure may also be influenced by dynamic factors such as the movement of air.
That is, upper atmosphere convergence can cause higher pressure at the surface.
Generalizations regarding high and low pressure at the surface:
Strongly descending air is associated with high pressure at the surface—a dynamic high.
Very cold surface conditions are often associated with high pressure at the surface—a thermal high.
Strong rising air is often associated with low pressure at the surface—a dynamic low.
Very warm surface conditions are often associated with relatively low pressure at the surface—a thermal low.
Mapping Pressure With Isobars
Barometer—instrument for measuring atmospheric pressure.
Millibar—an “absolute” measure of pressure, consisting of one-thousandth part of a bar, or 1000 dynes per square centimeter; equals 0.0147 pound per square inch.
Average sea-level pressure is 1013.25 millibars.
Isobar—a line joining points of equal atmospheric pressure.
Highs and Lows—are relative conditions, with the distinction depending on the pressure of the adjoining areas.
Ridge—an elongated area of relatively high pressure.
Trough—an elongated area of relatively low pressure.
Pressure Decreases With Altitude—on most pressure maps, pressure readings are adjusted to represent pressures at a common elevation, usually sea level.
Pressure gradient—the horizontal rate of pressure change, representing the steepness of the pressure slope; it has a direct effect on the speed of wind.
The Nature of Wind
Wind—horizontal movements of air; involve more area than do vertical motions.
Updrafts and downdrafts—small-scale vertical motions.
Ascents and subsidences—large-scale vertical motions.
Direction of Movement
Depends on the interaction of three factors:
Pressure Gradient force causes winds to move from areas of high pressure to areas of low pressure. The Coriolis Effect (Earth’s rotation)
Coriolis effect—the apparent deflection of free-moving objects to the right in the Northern Hemisphere and to the left in the Southern Hemisphere in response to the rotation of Earth.
Geostrophic wind—a wind that moves parallel to the isobars as a result of the balance between the pressure gradient force and the Coriolis effect.
Friction, or the frictional force of Earth’s surface, slows wind movement and reduces the influence of the Coriolis effect.
Wind Speed
Is determined by pressure gradient.
The steeper its slope, the faster the wind.
Describing wind speed — Frequently described in knots (nautical miles per hour).
Knot = 1.15 miles per hour, or 1.85 kilometers per hour
Global Variations in Wind Speed
- Surface winds are relatively gentle.
- Most persistent winds are usually in coastal areas or high mountains.
Wind speed usually increases with height.
Cyclones and Anticyclones
High-Pressure Wind Patterns — Anticyclone—high-pressure cell.
Low-Pressure Wind Patterns — Cyclone—low-pressure cell.
Eight circulation patterns are possible because of the interaction of the pressure gradient, the Coriolis effect, and friction.
Four involve anticyclones.
Four involve cyclones.
Each is dependent on the cell’s location (hemisphere and altitude [whether the surface layer or upper air]).
Vertical Movement Within Cyclones and Anticyclones
An anticyclonic pattern is upper air sinking down into the center of the high and diverging near the surface.
A cyclonic pattern is air converging at the surface and then rising.
The General Circulation of the Atmosphere
Rotation of Earth and its variable surfaces are key in creating a complex circulation pattern for atmosphere.
Only the tropical regions have a complete vertical cell.
The Hadley Cells—complete vertical circulation cells in which warm air rises to elevations of about 50,000 feet (15 kilometers), where it cools and moves poleward, then subsides. The cell’s air rises at the equator and descends at about 30° of latitude (either north or south, depending on the cell).
There are two.
At midlatitudes and high latitudes, vertical cells do not exist or are weakly and sporadically developed.
Seven Components of the General Circulation Pattern
- The general circulation of the atmosphere has seven surface components:
(1) Intertropical Convergence Zone
Intertropical Convergence Zone (ITCZ)
Intertropical Convergence Zone (ITCZ)— a belt of calm air where the northeast trades and southeast trades converge, generally in the vicinity of the equator.
Also called an equatorial front, intertropical front, and doldrums.
Front—a zone of discontinuity between unlike air masses.
ITCZ’s thunderstorms provide the updrafts by which all the rising air in the tropics ascends.
Often appears as a narrow band of clouds over oceans, but it is less distinct over continents.
(2) Trade winds
Trade Winds
Trade winds—the major wind system of the tropics, issuing from the equatorward sides of the subtropical highs and diverging toward the west and toward the equator.
Most reliable of all winds, being extremely consistent in both direction and speed.
Winds are named for the direction from which they blow.
Trade winds’ origin depends on which hemisphere they are in.
In the Northern Hemisphere they originate in the northeast, so they are sometimes called “northeast trades.”
In the Southern Hemisphere they originate in the southeast, so they are sometimes called “southeast trades.”
Warming, drying winds capable of holding enormous amounts of moisture.
Do not release moisture unless forced by a topographic barrier or pressure disturbance.
Pass over low-lying islands, which thus are desert islands.
Windward slopes in trade winds, as in Hawaii, are some of the wettest places on Earth.
(3) Subtropical highs
Subtropical Highs
Subtropical highs (STHs)—large, semipermanent high-pressure (anticyclone) cells centered at about 30° latitude over the oceans; they have average diameters of 3200 kilometers (2000 miles) and are usually elongated east–west. They develop from the descending air of the Hadley cells.
Horse latitudes—areas in the subtropical highs characterized by warm, tropical sunshine and an absence of wind; created because weather within an STH is nearly always clear, warm, and calm due to the fact that descending air does not form clouds and therefore cannot produce rain.
STHs also coincide with most of the world’s major deserts.
STHs serve as source for two of the world’s three major surface systems:
Trade winds
Westerlies
(4) Westerlies
The Westerlies
Westerlies—the great wind system of the midlatitudes that flows basically from west to east around the world in the latitudinal zone between about 30° and 60° both north and south of the equator.
Jet Streams
Two cores of high-speed winds at high altitudes in the westerlies:
Polar front jet stream
Subtropical front jet stream
Rossby Waves—sweeping north–south undulations that westerlies frequently develop in upper air.
Rossby waves and the migratory pressure systems and storms associated with westerly flow make the midlatitudes have more short-run weather variability than any other place on Earth.
(5) Polar front (subpolar lows)
A polar front is sometimes clearly visible by the semipermanent zones of low pressure called the subpolar low.
A zone of low pressure that is situated at about 50° to 60° of latitude in both the Northern and Southern Hemispheres and which often contains the polar front.
Characteristics vary in either hemisphere because the continents interrupt the northern subpolar system, whereas the southern is virtually continuous over the oceans.
Polar front—the meeting ground of the polar easterlies’ cold winds and the westerlies’ warm winds.
(6) Polar easterlies
Polar easterlies—a global wind system that occupies most of the area between the polar highs and about 60° of latitude.
The winds move generally from east to west and are typically cold and dry.
(7) Polar high
Polar high—a high-pressure cell situated over either polar region.
Because it forms over an extensive, high-elevation, very cold continent, an Antarctic high differs greatly from an Arctic high.
An Antarctic high is a strong, persistent, and almost a permanent feature, whereas an Arctic high is much less pronounced and more transitory.
Vertical Patterns of the General Circulation
Winds in upper elevations of the troposphere are different from surface winds.
The most dramatic difference occurs between surface trade winds and the upper-elevation antitrade winds.
Antitrade winds—tropical upper-air winds that blow toward the northeast in the Northern Hemisphere and toward the southeast in the Southern Hemisphere.
Modifications of the General Circulation
The general circulation varies because of many factors, but the two principal modifications are seasonal variations in location and monsoons.
Seasonal Variations in Location
The seven surface components of the general circulation shift latitudinally with the changing seasons.
Affect weather only minimally in equatorial and polar regions, but significantly alter weather in the midlatitudes and their fringes.
Monsoons
Monsoon—a seasonal reversal of winds; a general onshore movement in summer and a general offshore flow in winter, with a very distinctive seasonal precipitation regime.
Most significant disturbance to the pattern of general circulation.
Offshore flow—wind movement from land to water.
Onshore flow—wind movement from water to land.
Control the climates of regions with more than half of the world’s population.
Origin of monsoons is still not understood, though there is increasing evidence that it is associated with upper-air phenomena, particularly jet-stream behavior.
Monsoons have an essential impact—their failure or even the late arrival of monsoonal moisture inevitably causes widespread starvation and economic disaster.
Localized Wind Systems – Lesser winds have a considerable effect on weather and climate on a localized scale.
Sea and Land Breezes
Cycle of sea breezes and land breezes is a common local wind system along tropical coastlines and somewhat in summer in midlatitude coastal areas.
Essentially a convectional circulation caused by differential heating of land and water surfaces.
Land breeze—local wind blowing from land to water, usually at night (and normally considerably weaker flow than that of a sea breeze).
Sea breeze—local wind blowing from sea toward the land, usually during the day.
Valley and Mountain Breezes
Daily cycle of airflow occurs with valley and mountain breezes.
Convectional circulation caused by differential heating of higher versus lower elevations.
Mountain air cools quickly at night, allowing cooler air to drain down the slope in the evening. Conversely, valley air heats more rapidly during the day, allowing warm air to move upslope during the day.
Valley breeze—an upslope flow during the day.
Mountain breeze—a downslope flow during the night.
Air drainage—the sliding of cold air downslope to collect in the lowest spots, usually at night; a modified form of mountain breeze common in winter.Katabatic Winds
- Katabatic wind—a wind that originates in cold upland areas and cascades toward lower elevations under the influence of gravity.
Air is cold and dense, usually colder than the air it displaces in its downslope flow.
Mistral—a cold, high-velocity wind that sometimes surges down France’s Rhone Valley, from the Alps to the Mediterranean Sea. It has considerable destructive power.
Similar winds are called bora in the Adriatic region and taku in Alaska.
- 4. Foehn and Chinook Winds
Chinook—a localized downslope wind of relatively dry and warm air, which is further warmed adiabatically as it moves down the leeward slope of the Rocky Mountains.
Called foehn when it occurs in Europe.
Santa Ana Winds—similar to chinook/foehn. Have a high speed, high temperature, and extreme dryness, and prompt wildfires.
El Niño–Southern Oscillation
El Niño—an anomalous oceanographic weather phenomenon of the eastern equatorial Pacific, particularly along the coast of South America.
Occurs when southeastern trades abnormally slacken or reverse direction, which triggers a warm surface flow, which displaces the cold, nutrient-rich upwellings that usually prevail on the surface. Disrupts productive offshore fisheries.
Was once believed to be a local phenomenon, but is now understood to be associated with changes in global pressure, wind, and precipitation.
Occurs every few years around Christmas (El Niño is Spanish for “the Christ child”).
Even though archeological and paleoclimatological records have indicated past El Niño phenomena, it was not until 1982–1983 that great attention was drawn to it.
In 1982–1983 (see page 131), the El Niño event brought on a series of weather events called then “the most disastrous in recorded history” (1500 human deaths, about $9 billion in damage, and vast ecological havoc):
Crippling droughts (in Australia, India, Indonesia, the Philippines, Mexico, Central America, and southern Africa)
Devastating floods (in western and southeastern United States, Cuba, and northwestern South America)
Destructive tropical cyclones (in Tahiti and Hawaii)
Die-offs of fish, seabirds, and coral (from abnormally warm water over a 13,000-kilometer [8000-mile] stretch of the equatorial Pacific).
In 1997–1998 another strong El Niño cycle occurred.
Property damage exceeded $9 billion and at least 1500 people died.
Normal Pattern
To understand the El Niño phenomenon, it is critical to understand normal pressure, wind, and ocean current patterns in the Pacific.
Dominance of subtropical highs in the eastern Pacific causes westward movement of the trade winds toward a low-pressure cell in the western Pacific.
Trade winds create frictional drag on the Pacific Ocean and create a westward-moving warm equatorial current.
The removal of surface water near the western coast of South America allows cold water to upwell.
This phenomenon is known as the Walker Circulation.
This is a closed convection cell, but in reality is probably more complex.
El Niño Pattern
Every few years, the normal pressure pattern changes.
High pressure develops over northern Australia, and low pressure develops to the east over Tahiti.
This reversal is known as the Southern Oscillation, which is a large-scale fluctuation in sea-level atmospheric pressure that occasionally occurs in the eastern and western tropical Pacific; caused by differences in water temperature.
When El Niño and Southern Oscillation coincide (called ENSO), unusual atmospheric and oceanic conditions are more frequent and more intense than when either event occurs alone.
Pressure reversal causes the trade winds to reverse direction, and this allows warm water from the western Pacific to backwash toward the eastern Pacific.
For many months before the onset of El Niño, the trade winds pile up warm water in the western Pacific, and then a bulge of warm equatorial water about 25 centimeters high moves eastward in a series of bulges known as Kelvin waves.
These waves can take 2 to 3 months to arrive off the coast of South America.
This causes the sea level to rise off the coast of South America as the warm water pools.
The thermocline boundary between near-surface and cold, deep ocean waters lowers.
This impedes the upwelling of cold water off of the coast, and thus causes temperatures off the coast of South America to rise.
This shift in the normal pressure pattern in the Pacific can cause increased precipitation in the deserts of Peru, droughts in northern Australia and Indonesia, decreased monsoon activity in South Asia, and more powerful winter storms in the southwestern United States.
La Niña
La Niña—unusually cold temperatures in the eastern equatorial Pacific.
Both El Niño and La Niña are extreme cases of a naturally occurring climate cycle that involves large-scale changes in sea-surface temperatures across the eastern tropical Pacific.
La Niña is not as predictable as El Niño.
Causes of ENSO
There is no clear “trigger” of ENSO. It is not clear whether the changes in the ocean temperature or the changes in the pressure and wind occur first.
The effects of ENSO are also not completely predictable.
Teleconnections
Some generalizations, however, can be made (e.g., floods are more likely to occur in California during El Niño years).
There is increasing recognition of El Niño connections with atmospheric and oceanic conditions outside of the Pacific.
These connections are known as teleconnections.
Energy for the Twenty-First Century: Wind Power
Humans have long used wind to convert to other forms of energy.
Sailboats, grain milling, water pumping.
First wind electrical turbine was invented in 1887.
Modern versions are much more efficient via the use of aerodynamic lightweight materials.
Further engineering improvements have created a significant decrease in the cost of wind power.
Lighter materials, larger blades and turbines, computer monitoring to maximize efficiency.
Current wind turbine blades turn 12–15 rpm.
Wind farms are typically located in regions where elevation is higher and vegetation is minimal.
Electrical generation via wind is a viable source of energy given that there is no ongoing fuel cost.
Wind power is currently less expensive than electricity generated via fuel oil and natural gas.
Not as inexpensive as coal yet.
Advantages of wind energy:
Wind is free.
No need to seek and extract fossil fuels.
No greenhouse gases are generated.
Disadvantages of wind energy:
Intermittent nature of wind.
Windiest places in North America tend to be the least populated, so energy has to be transported over long distances.
Some argue that wind turbines are a form of visual blight.
Global Environmental Change: Changes in the South Asian Monsoon
Summer monsoon in India is critical to agriculture.
Normally first occurs in June, moving eastward and northward, and is over by the end of October.
When rains are late, crops fail.
When rains are overabundant, crops drown.
Evidence strongly suggests that global changes will substantially alter the South Asian summer monsoon.
Scientists have researched monsoon variability for more than a century.
They have discovered teleconnections with the strength and variability of ENSO.
Key to forecasting ability has been the increased availability of surface and satellite weather observations.
Changes to summer monsoon are likely to vary in magnitude among various parts of India.
Models show that over most of the country, monsoon rains will decrease and onset will be delayed.
Breaks in precipitation will become more common.
Disturbances will move more slowly, increasing the likelihood of flooding and decreasing precipitation totals.
Summer rains are expected to fail (40–70% of today’s norm) every 5 years.
Food security is greatly threatened by these changes.
India’s Meteorological Department closely monitors monsoons.
Mitigation policies are hard to implement because of the importance of the current year’s food supply.
Focus: Multiyear Atmospheric and Oceanic Cycles
Several long-term atmospheric and oceanic cycles have been identified in addition to El Niño.
The Pacific Decadal Oscillation (PDO) is a long-term cycle of sea-surface temperature change in the Pacific Ocean.
Cycles last up to 30 years but can shift abruptly.
During the “positive,” or “warm” phase, the eastern tropical Pacific is relatively warm and the northern/western tropical Pacific is relatively cool.
During the “negative,” or “cool” phase, the pattern switches.
Causes are not well understood, but changes in water temperature seem to influence the location of the jet stream and storm tracks across North America.
The North Atlantic Oscillation (NAO) is an irregular “seesaw” of pressure differences between the Icelandic Low and the Azores High.
In the “positive” phase, the Icelandic Low exhibits lower-than-usual pressure, while the Azores High exhibits higher-than-usual pressure.
In the “negative” phase both the Icelandic Low and Azores High are weaker.
The Artic Oscillation (AO) alternates between warm and cold phases that are closely associated with NAO.
During the “warm” phase (associated with the NAO positive phase) the polar high is weaker.
Cold air masses don’t move as far south and sea-surface temperatures tend to be warmer in Arctic waters (although Greenland tends to be colder than usual).
During the “cold” phases (associated with the NAO negative phase) the polar high is strengthened. Cold air masses move farther south and Arctic water is colder (although Greenland tends to be warmer than usual).
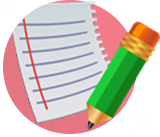
Accounting Homework
Stuck with a homework question? Find quick answer to Accounting homeworks
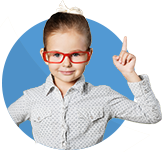
Ask Accounting Tutors
Need help understanding a concept? Ask our Accounting tutors
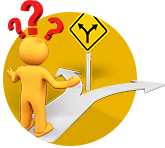
Accounting Exams
Get access to our databanks of Discussion questions and Exam questions
How We Safeguard Your Tutor Quality
All tutors are required to have relevant training and expertise in their specific fields before they are hired. Only qualified and experienced tutors can join our team
All tutors must pass our lengthy tests and complete intensive interview and selection process before they are accepted in our team
Prior to assisting our clients, tutors must complete comprehensive trainings and seminars to ensure they can adequately perform their functions
Interested in becoming a tutor with Online Class Ready?
Share your knowledge and make money doing it
1. Be your own boss
2. Work from home
3. Set your own schedule
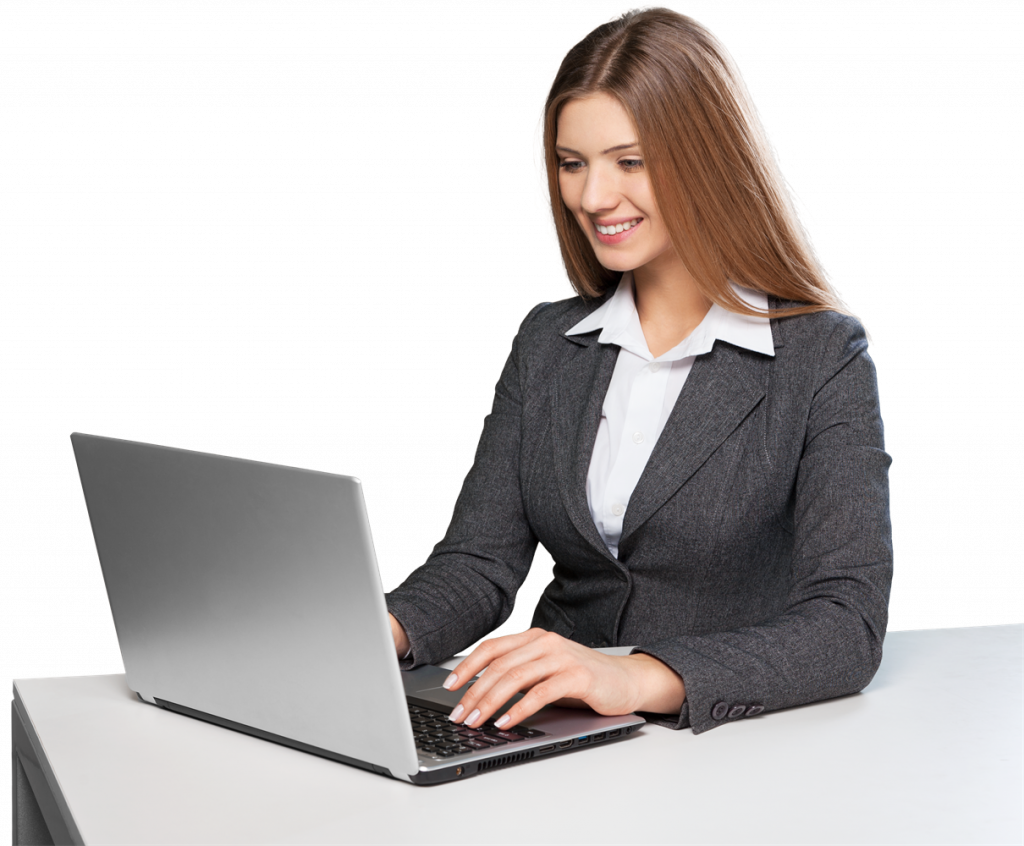
Geog 001
1. Introduction to Earth